Harnessing Molecular Forces in Engineering
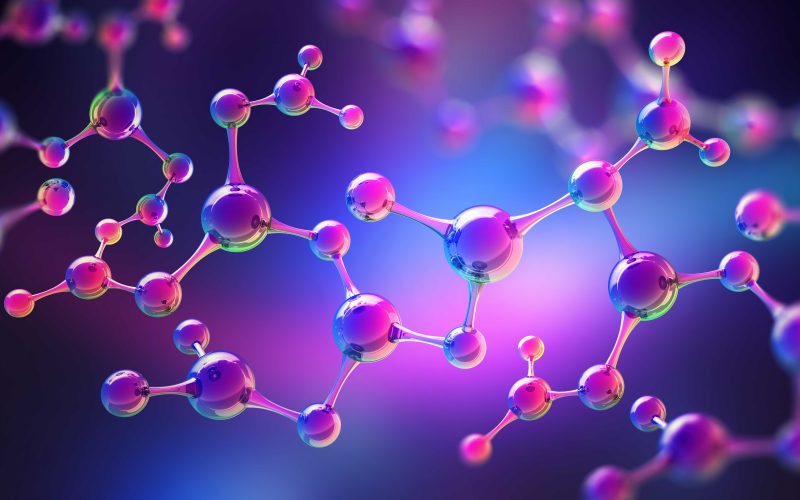
Nanotechnology, a field that deals with materials and devices on the nanometer scale, heavily relies on understanding and manipulating molecular forces. At this scale, molecular forces become predominant, governing the behavior and properties of materials. Scientists and engineers leverage these forces to design and fabricate nanomaterials with tailored properties.
One of the key applications of molecular forces in nanotechnology is in the synthesis of nanoparticles. By controlling the interactions between precursor molecules, researchers can induce self-assembly processes, leading to the formation of nanoparticles with precise sizes, shapes, and compositions. These engineered nanoparticles find applications in various fields, including electronics, medicine, and environmental remediation.
Furthermore, molecular forces enable the self-assembly of nanostructures. Through a process akin to molecular Lego, molecules can organize themselves into intricate patterns and structures, guided by the inherent molecular interactions. This self-assembly capability is exploited in the fabrication of nanodevices such as nanoscale sensors, actuators, and drug delivery systems.
Types of Molecular Forces
Van der Waals forces
Hydrogen bonding
Ionic bonding
Covalent bonding
Biomedical Engineering
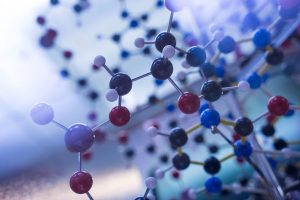
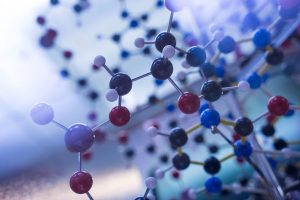
In biomedical engineering, harnessing molecular forces is essential for developing advanced medical technologies and therapies. One of the prominent applications is in drug delivery systems. By engineering drug molecules to interact with specific molecular targets in the body, researchers can enhance drug efficacy and reduce side effects. Molecular forces, such as hydrogen bonding and hydrophobic interactions, play a crucial role in the design of drug carriers and their interactions with biological tissues.
Moreover, molecular forces govern biomolecular interactions, which are fundamental to various biological processes. Understanding these interactions at the molecular level enables researchers to design biomaterials for tissue engineering, diagnostic assays, and targeted therapies. For instance, materials with specific binding affinities to proteins or cells can be engineered to promote tissue regeneration or selectively deliver therapeutic agents to diseased tissues.
Chemical Engineering
Chemical engineers harness molecular forces to design and optimize chemical processes for various industrial applications. Catalysis, the acceleration of chemical reactions by catalysts, relies on molecular forces to facilitate the formation and breaking of chemical bonds. Catalysts exploit specific molecular interactions to lower the activation energy required for reactions, thereby increasing reaction rates and improving process efficiency.
Similarly, molecular forces play a crucial role in separation processes such as distillation, adsorption, and membrane filtration. By exploiting differences in molecular interactions between components in a mixture, engineers can selectively separate and purify desired products from complex feedstocks. These separation techniques find applications in industries ranging from petroleum refining to pharmaceutical manufacturing.
Mechanical Engineering
In mechanical engineering, understanding and controlling molecular forces are vital for improving the performance and durability of mechanical systems. For example, in the design of lubricants and anti-wear coatings, molecular interactions between lubricant molecules and metal surfaces determine friction and wear resistance. By tailoring these interactions, engineers can develop lubricants that reduce friction and prolong the lifespan of mechanical components.
Furthermore, molecular forces influence the properties of materials used in mechanical engineering applications. For instance, in materials science, researchers study how molecular interactions affect the mechanical behavior of materials under different loading conditions. This knowledge informs the design of lightweight and high-strength materials for aerospace, automotive, and structural applications.
Electrical Engineering
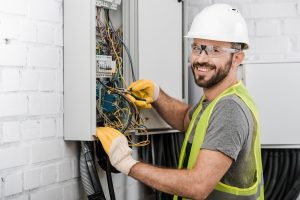
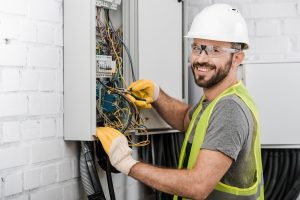
In electrical engineering, molecular forces influence the behavior and performance of electronic materials and devices. Dielectric materials, which are essential components of capacitors, insulators, and electronic devices, exhibit specific electrical properties due to molecular polarization and alignment under an electric field. Understanding and controlling molecular interactions in dielectric materials are crucial for optimizing their electrical performance and reliability.
Similarly, molecular forces play a significant role in semiconductor devices such as transistors and diodes. The electronic properties of semiconductors, including conductivity, mobility, and bandgap, are influenced by the arrangement and bonding of atoms in the crystal lattice. Engineers manipulate these molecular interactions through doping, surface treatments, and device geometries to tailor the electrical behavior of semiconductors for specific applications.
Environmental Engineering
Environmental engineers leverage molecular forces to develop innovative solutions for environmental challenges such as pollution remediation and water treatment. For example, in the field of environmental nanotechnology, nanoparticles functionalized with specific molecular groups are employed to capture and degrade pollutants in soil and water environments. The interactions between pollutant molecules and functionalized nanoparticles enable efficient removal and remediation of contaminated sites.
Furthermore, molecular forces play a crucial role in membrane-based separation technologies used in water treatment processes. Membranes with tailored pore sizes and surface chemistries selectively remove contaminants from water streams through mechanisms such as adsorption, sieving, and molecular sieving. These membrane-based technologies offer sustainable solutions for producing clean water and reducing the environmental impact of industrial processes.
Future Perspectives
Looking ahead, the field of molecular engineering holds immense promise for addressing global challenges and driving technological innovation. Advances in nanotechnology, materials science, and biotechnology are expected to further expand the scope of applications for harnessing molecular forces in engineering. From developing novel materials with unprecedented properties to revolutionizing healthcare and sustainable energy technologies, the possibilities are vast.
Challenges and Limitations
Despite the significant progress made in harnessing molecular forces for engineering applications, several challenges and limitations remain. One of the primary challenges is achieving precise control at the nanoscale, where quantum effects and surface phenomena become dominant. Developing robust experimental and computational techniques for manipulating molecular interactions with high precision is essential for realizing the full potential of molecular engineering.
Another challenge is ensuring the biocompatibility and environmental sustainability of engineered materials and devices. As the complexity and sophistication of engineered systems increase, the potential risks to human health and the environment also need to be carefully evaluated and mitigated. Ethical considerations regarding the responsible use of technology and the equitable distribution of its benefits are also paramount in guiding the development and deployment of molecular engineering solutions.
Ethical Considerations
As with any transformative technology, the ethical implications of harnessing molecular forces in engineering must be carefully considered. While these technologies offer unprecedented opportunities for innovation and progress, they also raise ethical concerns related to safety, equity, and privacy. It is essential for researchers, engineers, policymakers, and society as a whole to engage in thoughtful dialogue and decision-making to ensure that the benefits of molecular engineering are realized in a responsible and equitable manner.
Conclusion
In conclusion, harnessing molecular forces is central to the advancement of engineering across various disciplines. From materials science and nanotechnology to biomedical engineering and environmental sustainability, the applications of molecular engineering are vast and diverse. By understanding and manipulating molecular interactions, engineers can develop innovative solutions to complex challenges and pave the way for a more sustainable and technologically advanced future.
FAQs
What are Molecular physics ?
Molecular physics are interactions between atoms and molecules that determine their behavior and properties.
How do Molecular physics impact engineering?
Molecular physics are essential for various engineering applications, influencing material properties, chemical reactions, and device functionalities.
Can Molecular physics manipulated for specific applications?
Yes, scientists and engineers can manipulate Molecular physics to achieve desired outcomes in areas such as nanotechnology, material science, and biomedical engineering.
What are some challenges in harnessing Molecular physics ?
Challenges include achieving precise control at the nanoscale, ensuring biocompatibility, and addressing environmental concerns associated with certain applications.
How do Molecular physics contribute to nanotechnology?
Molecular physics play a critical role in nanotechnology by enabling the synthesis of nanomaterials, self-assembly processes, and the functioning of nanodevices.